Precision medicine – cutting edge healthcare
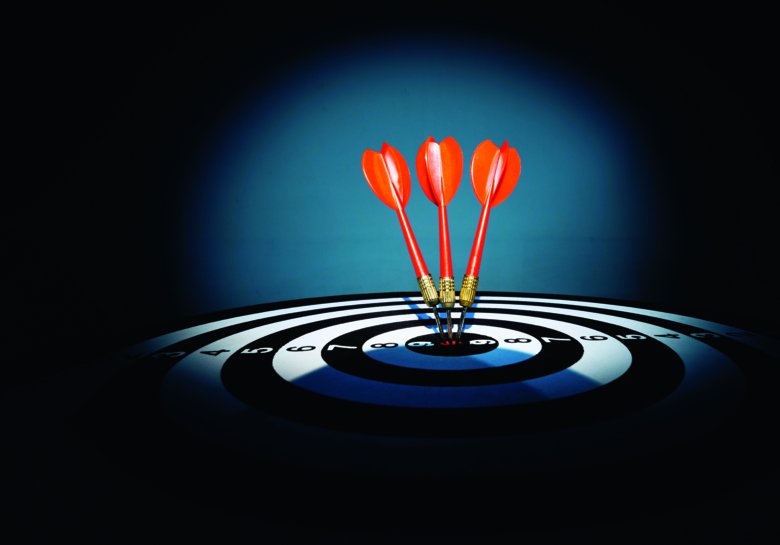
Not just care, but not just research either. No, precision medicine requires healthcare and research to find new ways of interacting. Meet the experts working at the cutting edge of healthcare. Read the first article in an in-depth series about precision medicine from KI's Swedish popular science magazine.
Text: Annika Lund, in translation from Swedish for the magazine Medicinsk Vetenskap no 4/2021 l Spotlight on Precision Medicine
SPOTLIGHT ON PRECISION MEDICINE. One thing needs to be said straightaway: precision medicine is not a precise concept. And it has different names, such as personalised, person-centred or tailored medicine. But – to describe it in imprecise terms – precision medicine is about finding out as much as possible about a patient in order to make the most accurate diagnosis possible and then select the best possible treatment. Or preventive measures. The term is, as we have said, not very precise.
And yes, this is of course what doctors have always tried to do. But in recent years, the volume of information that can be obtained about an individual patient has exploded. These days, it is technically possible to examine the entire genome of an individual person within the course of a day – which our healthcare system will do in urgent cases, for example, if an acutely ill child is suspected of having a congenital genetic illness. But normally results take a few weeks and the cost is about SEK 30,000, including the cost of interpretation.
The technology is here
Sweden has had the equipment for analysing, or sequencing, genomes for over ten years. Sequencing the entire genome has been possible since 2015. This powerful equipment is held at SciLifeLab, a research centre located on the university campus around Karolinska Institutet. Before they existed, it took years to analyse the genome of an individual person, at a cost that precluded everyday clinical use.
Alongside this advance in diagnostic technology, a series of targeted treatments requiring gene analysis have come to the market. It is now often possible to do what former US president Barack Obama noted in the context of an investment in precision medicine in 2015 – we can give the right medicine to the right patient at the right time.
In some areas of medicine at least. Rare congenital diseases, cancers, and infectious diseases are amongst the areas at the forefront of developments. There are several reasons for this – with rare congenital diseases, for example, often only one gene or a few genes are significant, and older technologies have already given us the option of analysing smaller numbers of genes. In addition, the tumour cells in blood cancers such as leukaemia and lymphoma often mutate less frequently, at least in comparision with many other forms of cancer. It is also easy to take samples for analysis for blood cancers. One blood sample is enough, which is less challenging than a biopsy of a solid tumour in the body.
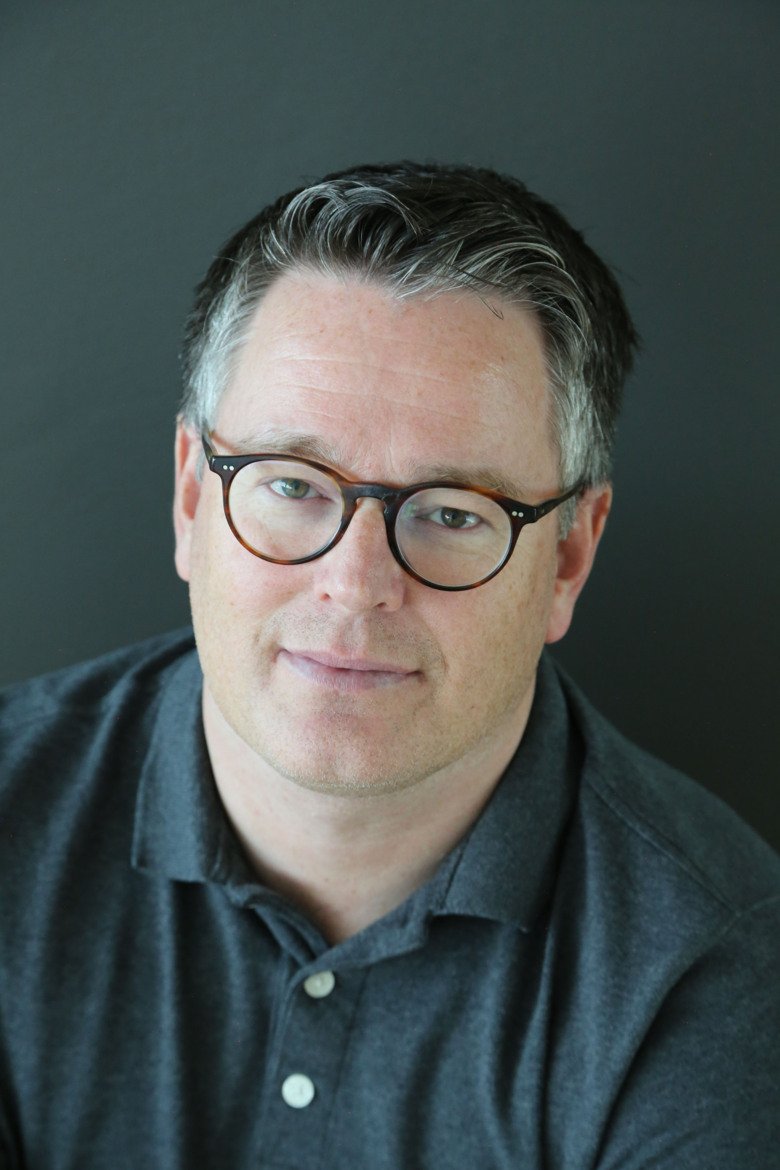
Already in use within hematology
“For decades, hematologists have been using different types of genetic analysis to divide diseases into subgroups and use these as the basis of treatment,” says Richard Rosenquist Brandell, Professor of Clinical Genetics in the Department of Molecular Medicine and Surgery at Karolinska Institutet. “The clinical benefits of gene analysis have been clear to us for some time. The time is now right to disseminate this way of working more widely within healthcare.”
One example is chronic myeloid leukaemia. We have known since the 1960s that the disease is due to genetic changes that lead to the formation of a protein that drives the disease. The early 2000s saw the arrival of a medicine that counteracts this protein, a tyrosine kinase inhibitor, which can now be taken in tablet form. As a result, this group of patients, whose prognosis was previously poor, has a life expectancy almost the same as people without the disease.
Achilles’ heel of cancer is found with gene sequencing
But blood cancers constitute only about ten per cent of all cancers. And even developments in terms of solid tumours are moving at breakneck speed. With several cancer types, it is now common practice to sequence the genes in the tumour and pinpoint the mutations in the lump itself. This has made it possible to create subgroups for every cancer type, where each subgroup has similar genetics – and those genetics become the Achilles’ heel for these tumours, weak points where they can be combated. There are already several medicines in use in the healthcare system that exploit these weak points in tumours. For example, there are certain breast cancer cells that, due to a genetic change, have a lot of HER2 protein on the outside of the cell. The protein is a signal to the tumour cells to divide and increase in number. For over 20 years, there has been a medicine that blocks this protein, causing significant disrupution to the tumour cells. It has radically improved life expectancy for the fifth of breast cancer patients who have HER2 positive breast cancer, whose prognosis was previously poor.
And there is now a booming market in targeted therapies. A long list of medicines requiring the patient (or the tumour) to be genetically examined are awaiting approval.
This was described in a report from the Swedish Agency for Health and Care Services Analysis in spring 2021. The report states that there are already around 70 medicines in use in the Swedish healthcare system that require genetic analysis. The absolute majority of these – almost 50 – relate to various types of cancer. There are also a number in the area of infection, including treatments for HIV and the acclaimed medicines for hepatitis C, for which it is essential to know which variant of the virus the patient has. There are also a number of medicines used for uncommon diagnoses, such as cystic fibrosis and hemophilia.
Great expectations
So what should we expect in the future? Well, according to rough estimates in the report, 300-400 new targeted medicines will have reached the market by 2030. On top of that, around 50 ‘advanced therapies’ are expected to be approved for use. According to the report, these are expected to relate to cancers, musculoskeletal diseases, skin diseases, kidney diseases, eye diseases and neurodegenerative diseases. Advanced therapies do not target anything specific in a cell or a gene; the treatment itself consists of cells, genes or tissues that have been substantially altered before being given to the patient. One example is CAR-T treatment, in which the patient’s own T cells are removed, grown and multiplied, and genetically modified. When they are then given back to the patient, they have learned to recognise a marker on the body’s tumour cells – so those cells become a target for the T cells.
There are great expectations for what is known as the ‘gene scissors’ – CRISPR – which make it possible to edit an individual’s genome. This opens the door to correcting very clearly defined individual mutations in somatic cells. There are currently clinical trials underway relating to several blood cancers, other cancers and inherited eye diseases.
In the future, the healthcare system also expects to see ‘tumour-agnostic’ medicines that can be used for several different types of cancer irrespective of the organ where the cancer originated. A number of these are already in existence. The first was approved within the EU in 2019 and applies to a change that has previously been discovered in about 30 types of cancer but only in certain patients within each disease group.
But – how can a treating doctor get their bearings in all of this? Well, this is where doctors in laboratory medicine are important. It is not reasonable to expect a treating doctor to send away a sample for gene analysis, and to then interpret the response alone.
“The more information we get about the patient and their illness, the more complicated it is to understand,” says Rosenquist Brandell. “So we need to work in teams, so that several professional groups can interpret the data that has been gathered and translate that into a decision about the right treatment. Pathologists, oncologists, geneticists, radiologists, molecular biologists and bioinformaticians – I can see there being several professions that will need to work more with each other to enable us to provide the right care to the right patient at the right time.”
But as new treatments are arriving in healthcare, new technological advances are being made in research. One area that may have a major role to play in our future healthcare is liquid biopsies. This is where a blood sample is taken to get material that can be genetically analysed – small fragments of DNA that can provide important clues. The method is already being used in prenatal diagnosis in Sweden, where a blood sample taken from a mother can show if the foetus appears to have a chromosome abnormality.
But cancer care services are also keen to start using this method, in the hope of being able to discover tumours long before they have started causing symptoms or in order to track responses to treatment. A trial is currently underway in the UK. There is also hope that liquid biopsies could be used for the early discovery, and, possibly, prevention, of conditions such as dementia, certain diabetes complications, eye problems and some kidney complications.
Healthcare and research pull each other along
This is in part still only research. But with precision medicine, the boundary between research and healthcare is less distinct – which is partly what is good about it, explains Rosenquist Brandell. The field of childhood cancer provides an example. A Swedish project is currently underway involving whole genome sequencing for all children who get cancer, in addition to genetic analysis of the tumour cells themselves. The idea is that the genetic analysis will deliver direct benefits by identifying those who might experience serious side effects from the medicines, in some cases identifying alternative treatments, and potentially providing an explanation for the cancer.
But the data that these analyses generate is also enormously valuable for our broader understanding of childhood cancer. Whole genome sequencing is also needed in research in order to provide better treatment options for children who have cancer in the future. It could be said that healthcare and research pull each other along – they use the same data first to diagnose and treat and then to research and develop even better treatments at the next stage. This interaction is more noticeable in precision medicine than in traditional healthcare, says Rosenquist Brandell:
“My colleagues and I strike a balance between research and healthcare. Only if something is clinically relevant should it be introduced into healthcare. But when research finds something that is clinically relevant, it must also be possible to quickly introduce it into day-to-day clinical activity.”
Rosenquist Brandell is the head of Genomic Medicine Sweden, a Swedish partnership initiative established to implement precision medicine in the healthcare system. Genomic Medicine Sweden was set up in 2017, and partners include universities, healthcare providers, industry and patient organisations. There are seven nodes across the country, located in places that are home to university hospitals. The initiative is funded by the participating partners and the government through Vinnova, the Swedish Innovation Agency.
“It’s important that everyone in Sweden gets the same access to these medical advances,” says Rosenquist Brandell. “People shouldn’t be advantaged because they live, say, in Stockholm where they happen to have a doctor with a particular interest in the subject. This is an issue I feel very strongly about, perhaps because I’m from Norrbotten province myself. Precision medicine requires new ways of working and we need to create procedures for this that can be introduced throughout the country.”
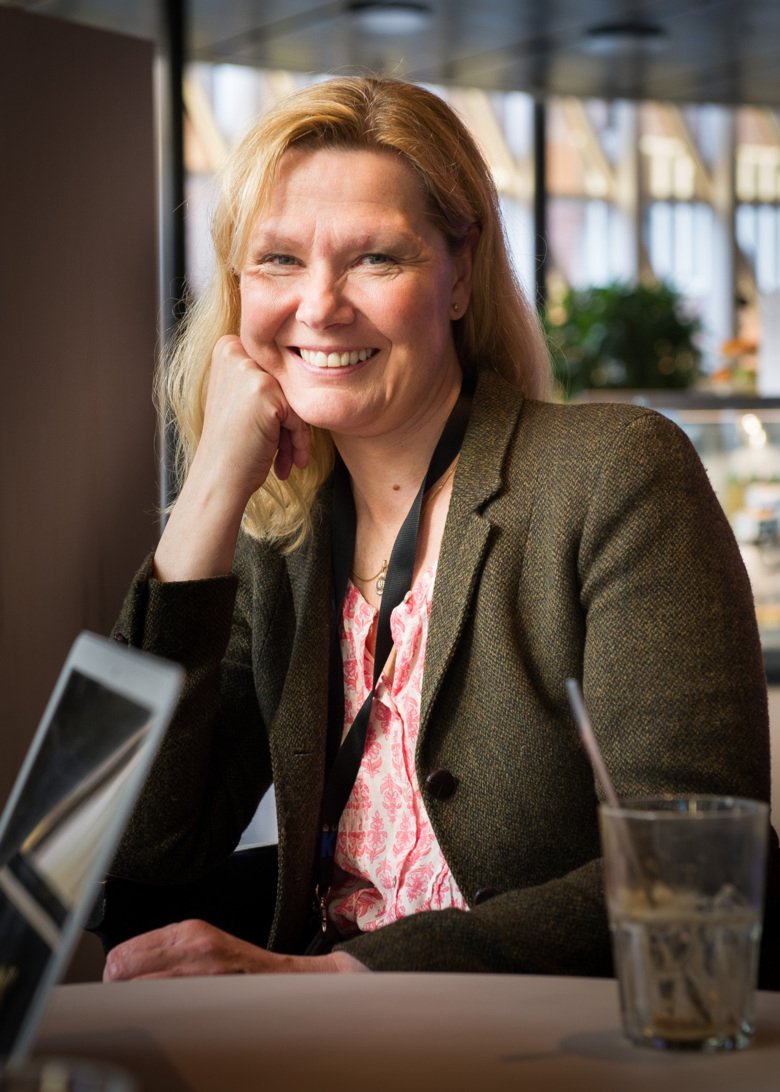
A natural way of thinking about rare diseases
One person fighting to get those procedures introduced into healthcare is Anna Wedell, Professor of Medical Genetics in the Department of Molecular Medicine and Surgery at Karolinska Institutet. She is clinically active at the Centre for Inherited Metabolic Diseases at Karolinska University Hospital, which cares for patients with specific rare diseases.
Wedell explains that it has long been natural for people working in the field of rare diseases to think along the lines that healthcare is now going in. One concrete example of this is the PKU test, which has been administered in Sweden since 1965 in order to identify the hundred or so children born each year with one of the 25 diseases currently covered by the screening. It means they then get early treatment and will not go on to develop serious illness.
Wedell and her colleagues started to perform large-scale gene analysis within healthcare at a very early stage. But the equipment belonged to the world of research, as it was located at SciLifeLab, which was set up to support large-scale research such as genomics. Meanwhile, the clinical benefits were clear – Wedell would encounter parents seeking help for their seriously-ill children who had symptoms that were difficult to interpret and no diagnosis. Performing whole genome sequencing on these children was giving them the chance of getting a diagnosis – and, possibly, treatment.
In a recently published study, Wedell and her colleagues summarise some of that work from the first five years at the centre where she works, the Genomic Medicine Center Karolinska – Rare Diseases (GMCK-RD). And of the more than 3,200 patients analysed, 1,285, or 40 per cent, got a diagnosis. The study describes these as transformative results that are game-changing for this group of patients.
And the group is said to be bigger than we might think. While each rare disease affects only a very small number of people, there are thousands of different diseases. It is estimated that up to six per cent of the population has a rare disease, in most cases genetic, with symptoms of varying severity. It may be that the percentage is even higher – in many cases, the disease is not discovered, so there is a great deal of under-diagnosis.
For some of these patients, many of whom are children, the treatment can be immediately life-saving or prevent the disease becoming severe. In other cases, there is no treatment. But a molecular diagnosis makes it possible to conduct research and, in the long term, perhaps develop a treatment. It also makes it possible for patients to seek each other out, perhaps via social media, and give each other day-to-day support to help manage specific difficulties.
Specialists from many different areas work together
Wedell describes the centre where she works, the GMCK-RD, as a bit of an oddity at the hospital. While what it does is certainly healthcare, its activity requires the use of equipment and expertise that are the province of reseach or of SciLifeLab. And in addition to that it needs specialists from many different areas, doctors and paediatricians, neurologists and endocrinologists, and laboratory physicians.
“We work together, in multidisciplinary teams,” says Wedell. “A clinical geneticist, for example, can identify an abnormal genetic variant. But a paediatrician will know that the protein that is formed is linked to epilepsy. It’s working in a team that enables diagnoses to be made.”
Staff at GMCK-RD are clearly already working in the way that is being seen as the way forward for precision medicine – laterally, between specialisms, in a close partnership with the research world.
So how has it been to work in that way?
Extremely stimulating – and extremely cumbersome, says Wedell. She describes the difficulties involved in basic things such as day-to-day legal and HR issues, funding and IT systems. How can SciLifeLab and healthcare share data? To what extent can you use staff employed at SciLifeLab within the hospital? How do you calculate the value of the care that is generated?
“There are major obstacles involved in working this way in healthcare,” says Wedell. “It’s possible to do it for a specific period, for example, for a research or developmental project. But if we want the model to be sustainable in the long term and also to be scalable so it can be introduced into more areas of medicine, we need to think again.”
Plans for a wider introduction
She is the head of the Precision Medicine Center Karolinska (PMCK), whose task is to help Karolinska University Hospital introduce precision medicine more widely. The first step was to create forums for staff at the hospital to pinpoint existing structural barriers. Two forums have been set up so far, one for cancer and another for rare diseases. Several others are on the starting blocks, for example for neurology, infection, rheumatology and psychiatry.
The plan is for precision medicine to be introduced more widely, including into work on the major endemic diseases. And throughout the whole of Sweden. The government’s 2019 national life sciences strategy states that Sweden aims to be a frontrunner in terms of introducing precision medicine into healthcare.
A working group within Genomic Medicine Sweden is to attempt to bring together those parties who want to make healthcare more personalised in the case of diseases such as diabetes, allergies, cardiovascular disease, dementia, Parkinson’s, MS and psychiatric and rheumatological disorders. In short – the aim is for the vast majority of those working in healthcare to now develop a precision medicine mindset.
Common diseases are complex puzzles
With the endemic diseases, the genetics are often more complex. There can be hundreds of genes involved, and each one may slightly increase the risk of getting the disease. So lifestyle and environmental factors play a major role, everything from our dietary, exercise and smoking habits to exposure to environmental toxins, exhaust fumes and stress at work.
It is a complex puzzle in which the route to developing a disease can be different for different people. And in many cases they also need to be treated differently. So what is precision medicine in this context? Surely not all Swedes with diabetes, an allergy or some other common illness can get treatment based on knowledge of their own genome?
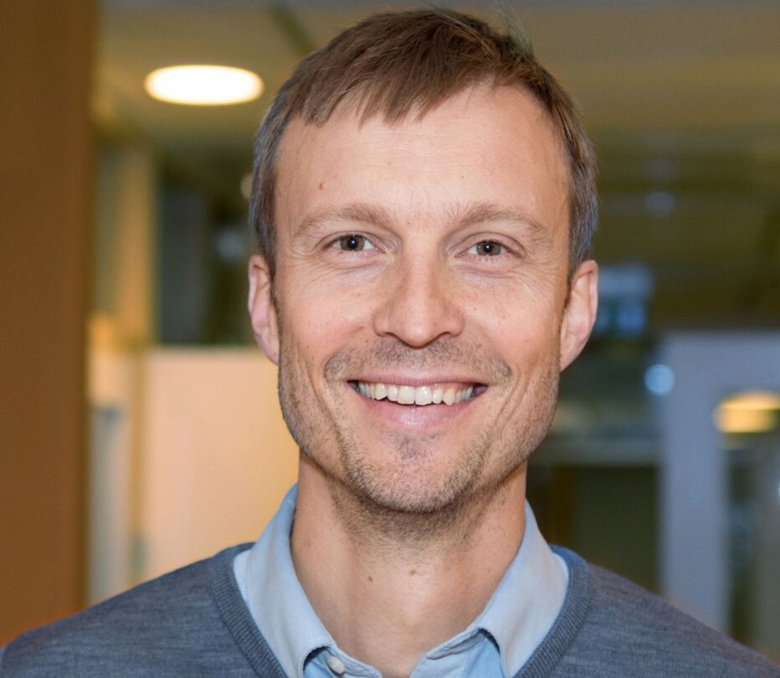
“One sensible approach might be to start with those people with the most serious illnesses where the clinical benefit is demonstrable or obvious,” says paediatric allergist Erik Melén, who until recently led the group at Genomic Medicine Sweden dealing with endemic diseases.
He takes an example from his own everyday experience. A baby was given emergency treatment for breathing difficulties, which could have been caused by a severe type of asthma but also by cystic fibrosis or another congenital disease. Following whole genome sequencing, a range of diseases could be discounted and doctors could very confidently diagnose severe asthma.
“That provided relief for the family and reassurance for me as a doctor that I was treating the right illness,” says Melén, who is also a professor in the Department of Clinicial Sciences and Education at Södersjukhuset hospital, Karolinska Institutet. “But we need a transparent discussion about how to put precision medicine into use across the whole country. To begin with, it may only be possible to do so in major centres of population, but how do we then disseminate the knowledge to smaller referring entities so that all patients get access to the same care?”
Another important issue in relation to endemic diseases is acquiring more precise tools so that the right treatment can be selected. Currently, many treatment ladders are based on starting with a tried and tested medicine and then working through the recommendations if the initial treatment has no effect.
This is where precision medicine has a major role to play, says Melén.
“We need treatment ladders in some form, but they now need to be designed and developed in other ways. This needs to be achieved through dialogue between clinical and genetic research and healthcare providers so that it becomes easier to select the right treatment immediately.”
Many “omics” will be knocking on healthcare’s door
But while the healthcare system is trying to implement genomics, technology is marching on. It is expected that a whole range of other “omics” will soon be knocking on healthcare’s door (see fact file). That may make it possible to get even more detailed information about an individual patient. They can be used, for example, to get an insight into different lifestyle and environmental factors.
These omics are a range of sources of very high-resolution information at varying stages of maturity. The real dynamite comes when they are combined. For example, you can investigate the exposome (our total environmental exposure) by using metabolomics (residues from substances we have been exposed to). You can then get a detailed picture of how urban environments, with exhaust fumes and noise, are associated with lung and vascular diseases.
This is the type of research project that Melén is currently involved in. It is an example of how omic data opens the door to a greater understanding of diseases in which the environment and lifestyle play a major role.
“A series of pilot projects are underway within healthcare in which various omics are being used and combined in various ways, so we will be getting several concrete examples of clinical benefit,” he says. “But we need a discussion about how far we should go with precision medicine – what patients is it appropriate for, how can we develop our testing and interpreting capacity and how can precision medicine be funded in the long term?”
Some large-scale data sources …
Genomics, the study of the whole genome (our approximately 22,000 genes) can provide information about what might happen to a person (i.e., various risks) and be used to provide a diagnosis. The genome can be compared with a reference genome that is considered ‘healthy’.
Epigenomics gives a picture of how the environment affects which genes are active or inactive.
Transcriptomics focuses on the genes that are currently active, i.e., the genes that produce RNA molecules and are active in the body’s protein factory.
Proteomics is about studying all the proteins in the body. In simplified terms, it can be said to reflect how everything that happens in the body, such as the development of disease, is made possible.
Metabolomics is the analysis of small molecules, such as various residues and degradation products. Reflects what has actually happened, such as various disease processes in the body or if someone has been exposed to substances such as food, drink or environmental toxins.
… and some major areas to be explored
The microbiome gives a picture of the several kilogrammes of bacteria normally within a body.
The exposome is the sum of all health-related environmental factors an individual is exposed to, i.e., everything that is not genes. It covers everything from how easy it is for a person to go for a walk around their local area to a family’s lifestyle and diet.
Sources: Richard Rosenquist Brandell, Erik Melén and others.
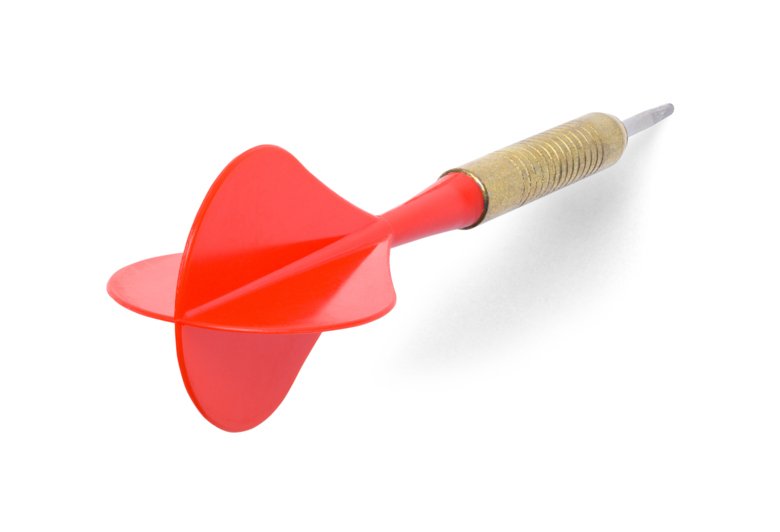
Targeting their research
Here are some examples of things that the researchers want to achieve with the help of precision medicine.
Better diagnosis of prostate cancer. With a PSA test, it is difficult to distinguish dangerous prostate cancer from harmless prostate cancer. If we instead analysed more protein markers and more than 200 genetic markers and took into account factors such as age and familial cancer, it would be easier to separate out prostate cancer that requires treatment.
Better prevention of cardiovascular disease. Genetic risk scores, which are calculated by weighing up information about a large volume of genetic variants and combining with traditional risk factors, have been shown to be a reliable way of identifying the individuals at greatest risk of cardiovascular disease, which opens up the possibility of putting more powerful preventive measures in place.
More effective cancer treatment. Genetic analysis is very important when selecting a cancer therapy. But alongside a description of the mutations that exist, the number of mututations matters too. Tumours with a very large number of tumours – a high mutation burden – can sometimes be more receptive to immunotherapy.
Smarter treatment of infection. Rapid analysis of bacteria makes it possible to select narrow-spectrum antibiotics – targeted antibiotics – even in urgent cases. Currently, broad-spectrum antibiotics are often used in urgent situations. But broad-spectrum antibiotics can increase the risk of antibiotic resistance and also have a greater effect on the body’s natural bacteria, which can cause more severe side-effects.
Fewer side-effects from medicines. It has been estimated that around ten per cent of the healthcare budget is used for managing side-effects. Genetic analysis can reveal if someone is at risk of serious side-effects, may not experience any effect, has a fast metabolism or is possibly over-sensitive to a particular medicine.
Reduced recurrence of stroke. Researchers hope that genetic analysis will increase the possibility of distinguishing between different types of stroke, which would make it easier to prevent recurrence.
Sources: Richard Rosenquist Brandell, Erik Melén, Läkartidningen (Swedish medical journal), the Swedish Agency for Health and Care Services Analysis and others.